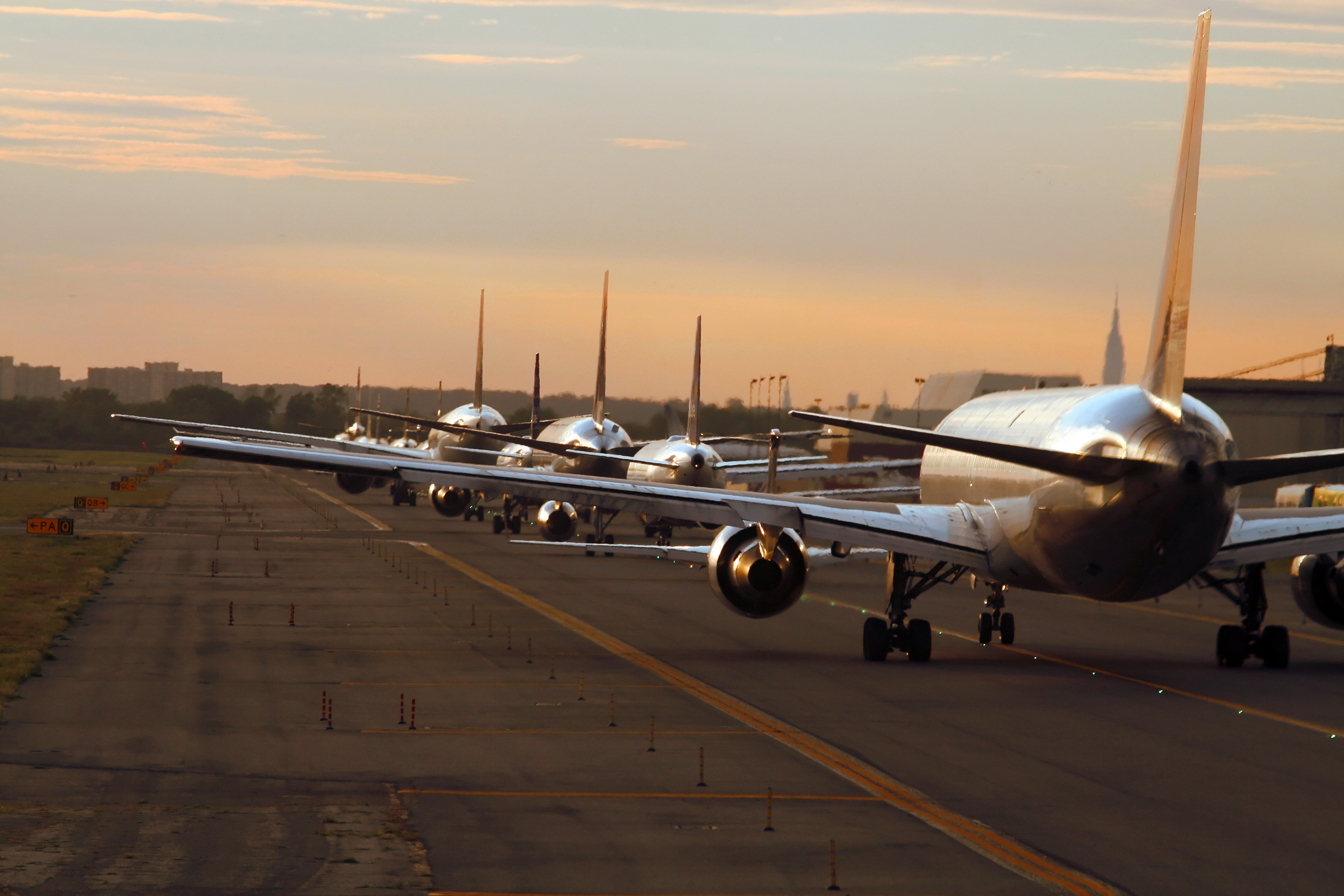
Sustainable Aero Lab Whitepaper
Bridging the Gap to 2050: How to Decarbonize Aviation Faster With Today’s Technologies
1) We need to talk about aviation!
Aviation is an industry that has transformed the world. It is an indispensable part of modern life and essential to social and cultural communication and understanding. Many of the advancements we have seen in the last 100 years would not have been possible without this industry.
Millions of people get on a plane every day to do business, go on holiday, help those in need, or visit their family and relatives. And the number of those who get on a plane continues to grow, despite the Covid-related dent. The International Air Transport Association (IATA)
forecasts that the global number of passengers on commercial flights will almost double by 2040. Already, one billion people are living in a diffe- rent country from their families today, creating a solid demand for transfer continents. With ongoing migration, this will only rise further. Still, most humans have never flown and strive to see the world. During the pandemic, we realized that many business trips were unnecessary, but on the other hand, we came to value the necessity of human connection. It means reduced business travel will be offset by increased leisure and social travel. People’s will to fly will increase, not decrease.
At the same time, aviation has a problem destined to become bigger and bigger: Further air travel growth means a further increase in emissions. As the world’s societies – especially Generation Z entering adulthood – become increasingly aware of climate change, emissions will become less socially acceptable. Unless we significantly decarbonize aviation and do this quickly, its current operating model will eventually become a dead- end.
More and more people involved with aviation have realized this in recent years. We have seen a return of the industry’s pioneer spirit in working on new technologies and the launch of exciting projects in electrification, sustainable fuels, and hydrogen as fuel. All these segments will be vital in bringing aviation forward and shaping the future of this industry. During its 77th Annual General Meeting, IATA approved a resolution for the global air transport industry to achieve net-zero carbon emissions by 2050. For this to happen, a minimum of 1.8 Gigatons (Gt) of carbon generated by a projected 10 billion passengers must be abated in 2050.
Solving aviation’s climate footprint will be the most significant technological achievement since the beginning of human flight. But to truly tackle the challenge, we will need a holistic approach that looks at the entire chain of sustainable aviation, from the cradle to the grave.
The electric car industry vividly shows us how everyone focuses on emissions “at the tailpipe” and forgets about other factors, such as the environmental footprint of mining the raw materials required for manufacturing batteries, as well as the impact generated by the energy production itself, which in most cases is not even accounted for in transportation statistics.
Being holistic also implies that, in a global industry, we must work together at different levels and on different solutions, aiming for the same shared goals. One thing that has already emerged is that electrification and hydrogen might be excellent solutions in the longer term but will not bring any significant leverage in the next 20 years (as shown in the following pages).
Albeit relatively small on the global scale, aviation’s share of global carbon emissions continues to rise. This means the industry cannot wait for technological silver bullets but double down on efforts to decrease its footprint with measures that can be applied today.
To contribute, we want to outline how we can use today’s technologies to decarbonize aviation within the next 20 years without neglecting aviation’s emerging new technologies.
1.1. Commerical aviation today
Let us dive deeper and examine how commercial aviation is being made up today.
2) Things We Learned About the Future
The world has already found common ground on decarbonizing road transport with electric vehicles. We know how to decarbonize energy production via wind, solar, and nuclear at a com- parable or lower cost to fossil fuels. Decarboni- zing industrial processes is at an earlier stage of development but also appears to be a solvable issue from a technical point of view.
Aviation, in contrast, is a tough nut. While the aforementioned other sectors are projected to decarbonize reasonably fast, aviation’s contribution to the global share of emissions is bound to rise much steeper than the recent growth trajectory suggests. At the same time, it is important not to insert an undesirable element of risk into what is today the safest mode of transportation by pushing technologies into the air that are not yet fully ready.
At Sustainable Aero Lab, we have been working with startups at the forefront of decarbonizing aviation for the past two years. We crystallized five major areas of development:
Hydrogen,
Sustainable aviation fuels,
New aircraft designs and materials,
Operational efficiencies,
Electrification.
Altogether, in the 24 months since our inception, we have mentored more than 40 startups worldwide, together with a who-is-who of experts from aviation, industries, entrepreneurship, and venture capital.
2.1 eVTOLs and battery-powered aircraft won’t solve our problems
A significant percentage of the aviation startups featured in the media come from the much-hyped eVTOL segment (short for electric vertical take-off and landing). This is the hyped ecosystem around novel air taxis, with Joby Aviation and Archer being among the best-known. Yet the longer we engage with startups, the more we realize that funding eVTOLs with a realistic range below 100 km has been a big distraction. eVTOLs do not decarbonize commercial aviation. They are an incremental activity, as they will replace no commercial aircraft from Airbus, Boeing, or other existing manufacturers.
For going beyond 100 km in the operations range, the required energy from batteries would make the aircraft too heavy. Electrification works for cars, where the combustion engine has a technical limitation of roughly 25% efficiency but where braking in traffic can reduce the overall system‘s efficiency to 5% of fuel energy used to propel the vehicle. The most modern airplanes, by contrast, convert 50% of the fuel energy into propulsion.
We have recently witnessed several startups developing full-electric electric aircraft converting to hybrid-electric propulsion. But even then, the technological gains are unproven. Swedish company Heart Aerospace, which has signed with several large airlines, including Air Canada and United, is the most prominent example. Other startups, such as Ampaire, have followed a hybrid-electric approach from the beginning, preparing a Cessna Caravan for the next round of test flights.
2.2 Hydrogen won’t help us – at least yet!
Another field with significant startup activity is hydrogen. The dream of hydrogen being used to store cheap wind and solar energy, and to be employed, for example, in industrial processes, is understandable, and so is the idea to use it for aircraft propulsion. California-based Universal Hydrogen, co-founded by former CTO of Airbus and UTC, Paul Eremenko, and UK-based ZeroAvia, are the two most renowned upstart companies in this sphere. Hydrogen is also the solution that Airbus has decided to invest in as a long-term solution, promising an airliner running on this fuel for 2035. At Sustainable Aero Lab’s SXSW panel in 2022, the company H3 Dynamics, founded by our mentor Taras Wankewycz, unveiled the first working hydrogen-powered nacelle, which flew with an uncrewed aerial vehicle later that year and can be scaled to turboprop-size.
Hydrogen-powered aviation can be split up into three main aspects:
-
There are two alternative solutions: convert today’s gas turbine engines to burn hydrogen or produce electricity in a hydrogen fuel cell that drives an electric motor. The burn solu- tion has drawbacks regarding non-greenhouse gas emissions but scales to 150-seat aircraft or larger. The fuel cell solution is emission-free, but available technology does not scale above 100 seats.
-
Hydrogen can be stored in a gaseous or liquid state. For implementation in commercial aviation, gaseous hydrogen will be too volu- minous. Liquid hydrogen is the solution chosen by all players. It requires more tank volumes than today’s kerosene and must be cooled down to -253°C. Yet liquid hydrogen offers unquestionable advantages compared to gaseous H2, making it the solution for aviation. However, this type of fuel‘s impact on aircraft design should not be underestimated. The tanks storing liquid hydrogen must be circular to keep cryogenic losses low. This brings the need to store tanks inside the fuse- lage – as circular-shaped tanks cannot be fitted in the wing, unlike today’s kerosene tanks of similar energy content. The result is a need for new aircraft architectures with in- creased overall volumes for the same passenger capacity. However, with only a marginally higher weight: hydrogen is lighter than jet fuel for the same energy content. Comparing fuels also has to take into account the correct metrics. Energy kJs are at the forefront, followed by design-impacting units like weight and volume.
-
Hydrogen will need to be supplied to the aircraft at the airport in parallel to the exis- ting infrastructure for kerosene. This will re- quire building an entirely new infrastructure that has yet to exist. The challenge would not necessarily have to be faced with the “pipe & truck” solution generally used for kerosene. For example, Universal Hydrogen aims to build a system of hydrogen fuel containers for aircraft that can be swapped like a baggage container while the plane is on the ground.
Hydrogen projects are being increasingly well- funded, pushed by the global wave of invest- ments supporting ESG goals. There is growing confidence that we could see the first commer- cial hydrogen aircraft fly and be certified before 2030. It will then be a sub-100-seat converted turboprop. However, this is different from the seg- ment where mass transportation is concentrated in terms of passengers or cargo. Addressing and solving all three aspects of hydrogen flight, in- cluding scaling the ground infrastructure, will take decades. It is plausible that hydrogen will be- come a solution for aviation in the long run, but not before 2050. Until then, it could serve us well as a base for sustainable aviation fuels.
2.3 The aircraft that fly in the future are already in production today
The world’s active commercial fleet consists of ca. 25,000 aircraft flying daily. Moreover, while we evaluate the “next big thing,” close to 2,000 further aircraft are leaving the production lines every year. Typically, airplanes will fly for 25 years before they are scrapped. And all commercial airplanes with over 100 seats built in the next 15 years will be conventional airplanes. This period will extend when looking at long-haul planes, burning about two-thirds of all fuels. With this in mind, it is easy to see that the aircraft models al- ready operating today will also make up the dominant part of the global fleet by 2050, needing today‘s infrastructure.
In aviation, technologies go through readiness levels (TRLs) to ensure high safety and security standards. But it only readies the aircraft and engines. Airports are nowhere near ready to cater to hydrogen and/or electric aircraft.
For significant technology changes, ground infrastructure on a global level will need to be adapted, too. Allowing new technology aircraft to be adequately operated takes considerable time. It will be crucial to run things in parallel. Other aspects, such as digitalization and improving air traffic control routings, novel aerodynamics, or lighter airframe materials, will all be complimentary – but will not yield a net-zero result.
Thus, we will add around 20,000 newly-built conventional planes in the next years. They will consume less energy than the aircraft in service today but will not deliver significant progress to reaching net-zero emissions and will fly beyond 2050. Even in a best-case scenario, we will not see substantial numbers of hydrogen or battery-powered aircraft in the segments that burn the brunt of Jet fuel in the next 25 years. To make it worse, all of aviation’s current planned “moonshot” projects – from eVTOL to hydrogen turboprop – are in the segment with 100 seats or less, the segment already irrelevant when looking at emissions. When working on breakthrough technologies, it makes perfect sense to start small. Yet, if we want to move the needle towards decarbonized flight before 2050, the answer can’t be a hydrogen or electric “moonshot” project.
2.4 Aviation’s growth will eat efficiency gains for breakfast!
Even if the latest aircraft technologies have lowered energy use, it won’t be fast enough to slow down emission growth. To illustrate this: the latest engine technology, which is used, e.g., on the Airbus A320neo and Boeing 737 MAX, has achieved a 15% reduction in fuel burn and, with this, emissions. This latest engine generation entered the market ca. 30 years after its predecessors. The stats look a little better for the generation before that, when moving from the Boeing 727 era to the A320 age resulted in 35% improvement, with 25 years in between (Part was moving from 3 to 2 engines, the rest were aero-dynamics, weight savings, and engine technology).
A Trip From NYC to LAX in 2040: A Tale About Aviation’s Dilemma
It is a beautiful spring day as you leave your hotel in Manhattan. The nice weather means you can take an electric air taxi from the Financial District to Newark Airport, which would not have been possible the day before when it was foggy. As you board the sleek-looking zero-emission eVTOL that seats seven passengers, the pilot informs you that takeoff will be delayed for another 20 minutes as there is still congestion around Newark’s main runways and its eVTOL vertiport. While the travel time from the hotel to the airport will thus not be any shorter than using the train or a rideshare, you still look forward to the scenic views on the short flight and to the superior passenger experience as you could already do your security check at the vertiport in Manhattan.
Finally, you have made it to the gate in Newark, and you look outside to watch the buzzing action on the tarmac. Newark is a hub airport for United Airlines. You can‘t spot any of their all-new hybrid-electric 30-seat turboprops from Swedish upstart Heart Aerospace, which has been part of the airline’s fleet for some years. But not here, as the heavy congestion at Newark would eat into the precious energy reserves of the aircraft, which is why the airline operates them primarily on thin routes, mainly in the Great Plains. You do, however, spot one of the new Airbus aircraft that was officially unveiled at the Paris Air Show in 2035 and flies on 100% hydrogen. Marveling at the world’s first fuel-cell aircraft in serial production, you would love to be a passenger on this futuristic plane today – but you are not, as it is a 78-seat turboprop with limited range and so far only operates to Vermont, a state which has heavily subsidized its airport infrastructure to support green hydrogen in the past three years. Unlike it was done in New Jersey, the state where Newark is located and where it needed a White House intervention to fund the build-up of any hydrogen infrastructure.
As the year is 2040 only, you comfort yourself that hydrogen-powered aircraft on commercial routes have only been operating for some years and that as the segment advances and adds range, it will be a different story by 2055. But, for your trans-con flight to LAX today, you are looking at an old friend: a 2031-built Boeing 737 MAX 10, the trusted workhorse of this route, which will bring you to the West Coast in just under 6 hours today.
Let’s reflect on the scenario above: We have just fast-forwarded 17 years in aviation and painted the best-case scenario, only to end with a trunk route being flown by the same aircraft family as today. Yes, eVTOLs would be cool, but they still compete with the less-emitting train to Newark and only add to global air traffic without lowering carbon emissions. Yes, hydrogen is an exciting future that can already be grasped in our story. But with rapidly developing climate change, we will not have 17 years to wait for the next revolution in aerospace to slowly become a reality. It is time to up the game.
We have become increasingly concerned that too much speculation about future technologies could cost us decades in lost opportunities to reduce carbon emissions and global warming in aviation dramatically.
3) What You Should Know About Sustainable Aviation Fuels (SAF)
3.1 What is SAF?
Sustainable aviation fuel is made from renewable sources, including used cooking oil, municipal waste, and woody biomass alongside electric energy (in the case of synthetic SAF). In addition to these sources, SAF can be derived from energy crops, such as fast-growing plants and algae. SAF is very similar in composition to traditional fossil jet fuel. Interestingly, SAF is a “drop-in fuel” which can be blended with fossil jet fuel. In turn, the blended fuel requires neither special infrastructure nor equipment changes. Given this, SAF can be shipped in the same pipelines as conventional jet fuel and adopted with minimal retrofit costs.
SAF is considered a safe, reliable fuel, certified by the jet fuel approval authority ASTM Interna- tional for blend ratios of up to 50% for existing jet engines and up to 100% for new engines.
Older engines need an aromatic component in the fossil jet fuel to swell the fuel system seals, which is why the blend ratio must stay below 50%. As aromatics are “unwanted” components in jet fuel (they burn incompletely and produce soot), they are not added to SAF, where the production process does not produce aromatics.
SAF can potentially reduce lifecycle emissions by up to 80% compared to conventional aviation fuel. This largely depends on the sustainable feedstock used, the production method, and the supply chain to the airport. SAF, as it is currently known, was first used by the aviation industry in 2008. According to the IATA, since 2016, more than 370,000 flights have taken off using a SAF contribution.
3.3 There are four ways to produce SAF
There are presently four main production methods, or pathways, for SAF:
1| HEFA: Short for „Hydroprocessed Esters and Fatty Acids.” It’s the classical oils and fats SAF and constitutes over 95% of today’s SAF. According to experts, the share of HEFA- based SAF is projected to stay above 80% by 2030, potentially covering 5%–10% of the total jet fuel demand. The feedstock is more significant than one thinks, but care has to be taken by the SAF industry to not compete with feedstock for food production. Excluding all edible oil crops, a reduction of greenhouse gas emissions of up to 84% can be achieved for this kind of SAF, compared to fossil jet fuel.
2| Alcohol-to-jet: This procedure converts biomass into ethanol, isobutanol, or methanol – and then into aviation fuel.
3| Gasification & Fischer-Tropsch is the biomass but, more importantly, the waste-based path-way. Here, carbon monoxide and hydrogen are gasified from the feedstock and then converted further into synthetic fuels. This happens in a chemical process called Fischer-Tropsch, named after the two German researchers who developed it in the 1920s. It is still in the commercial pilot phase and will have little importance until 2030, but it has long-term potential. Feedstock potential is vast, but the waste collection process as input for the pyrolysis to syngas is fragmented and will have to be established, too. Compared to fossil jet fuel, methods 2) and 3) are believed to reduce greenhouse gas emissions by up to 94%.
4| Power to Liquid (PtL), or e-fuel: A long-term source that will require cheap energy that can’t easily be routed to consumers to motivate this pathway. Direct capture of solar energy and production of SAF in reactors would pose a long-term possibility – feedstock that is, in theory, available in abundance. Until 2030 and possibly until 2050, this is projected to remain a low-volume path because of the energy cost. A proof of concept is also not realistic until the late 2020s. The PtL method is believed to have the highest potential of reducing greenhouse gas emissions of all SAF methods, nearing 100%.
3.2 What makes SAF sustainable?
SAF does not reduce “tailpipe” emissions from burning fuel in-flight. The calculated reductions in greenhouse gas emissions come from the life cycle of jet fuel.
Consuming conventional jet fuel releases fossil CO2, pulling it from the ground and adding it to the biosphere‘s total carbon. Meanwhile, burning SAF returns carbon to the atmosphere that had previously been absorbed by plants or that would have been released as industrial waste gasses or household garbage.
The result is a net “well-to-wake” reduction in life-cycle CO2 emissions. This can be as high as 80% for the SAF produced from biomass (e.g., cooking oil). As a result, commercial flights powered by SAF are coined “net-zero,” as opposed to “zero emissions.” The latter would only be possible through zero emissions at the tailpipe, as in the (projected) case of a hydrogen-powered aircraft using completely green hydrogen and releasing the hydrogen energy in a fuel cell that drives electric motors. If the hydrogen is burned in a gas turbine instead, there is no release of CO2 either, but we’ll still see NOx emissions, albeit at a lower level than for jet fuel.
Synthetic fuels produced from captured CO2 and renewable electricity can reach well-to-wake reductions of almost 100% relative to fossil jet fuel. Some feedstocks even promise to be carbon negative.
Researchers at the US National Renewable Energy Laboratory report that SAF made from food waste has the potential to reduce net carbon emissions by upwards of 165%. This reduction claim is based on preventing food waste rotting in landfills from releasing methane, a greenhouse gas 20 times more potent than CO2.
3.4 Scaling SAF production – Which timelines are realistic?
When talking about SAF, it is essential to understand the volumes. The global consumption of jet fuel was around 300 million tons in 2021. The world’s production of SAF was 0.2 million tons. In other words, less than 0.1% of the consumed fuel. Experts think we could reach a share of up to 8% by 2030 if properly talked about and if planned investments are being made. The ramp-up of the different production methods is also different. The following chart shows the expected volume growth between the different SAF production methods.
HEFA will dominate SAF production past 2030, but then other methods should catch up. It depends on the cost of production, which is pictured in the report we used for the other figures, as in the following chart.
To scale SAF production as rapidly as in the shown projections, governments must introduce incentives for investing in production facilities. Increasing quotas of SAF percentages would create such an environment.
Several airlines and manufacturers, like Airbus, have set a target to reach a 10% SAF blend level by 2030. Given that production volumes will be challenged to reach 10% of the annual fuel volume, this target will only be achieved with significant uptake and production of sustainable fuels.
3.5 In a nutshell – Why SAF is so pivotal to aviation at this stage:
1| We already know how to produce sustainable aviation fuels. It is a question of scaling, not a question of technology.
2| While no aircraft is yet(!) certified to fly on 100% SAF, it is relatively easy to start by blending more SAF into the existing fuel mix. Increasing the percentage of SAF in the blend now would have a much more significant im- pact than only going for a 100% SAF fuel option later.
3| Using SAF means fully utilizing the existing airport infrastructures, from pipes to fuel trucks and apron installations.
4| SAF can be used as a drop-in replacement for current fossil fuels. Therefore, existing engines don’t require modification.
5| Producing more SAF and blending it into the existing fuels is an applicable measure that can be easily supported by any government or regulators worldwide.
4) How We Can Reduce Aviation Emissions Today
We have identified three pathways to effectively drive down emissions in commercial aviation in the next 20 years.
4.1 PATHWAY #1: Accelerate the introduction of Sustainable Aviation Fuels (SAF)
In the last chapter, we focused so heavily on SAF because scaling its production is the most critical lever to lower commercial aviation emissions until 2050.
“SAF, if made from waste or synthetically, is probably the only viable solution to dramatically decrease the carbon emissions for aviation in the next two decades. The challenges in supplying truly sustainable fuels remain gigantic with scaling production and lowering cost”, says Sustainable Aero Lab CEO Stephan Uhrenbacher.
The capabilities of production and the maturity of technologies will limit the acceleration of SAF. To increase the share of SAF as efficiently as possible, we will have to concentrate on the most mature method first, which is HEFA, and work to mature the others as quickly as possible. This means that SAF will remain a field of utmost relevance both politically and investment-wise.
4.2 PATHWAY #2: Reintroduce more turboprops
In the late 1990s, we saw regional jets killing most of the demand for turboprops despite their eco- nomic advantages and despite turboprop aircraft becoming more comfortable for passengers since then. In the last 15 years, we saw another shift towards larger jets with lower seat-mile costs (except on US routes where scope clauses still apply). But these larger aircraft need partly induced demand to fill them and to operate in economically viable ways.
This leaves us with a very limited market for turboprop airplanes today regarding availability and seats. With the comfort levels that modern turboprops can offer and potential faster travel times for distances up to 600kms due to more optimal flight routes, there is an obvious benefit in building new turboprop designs with the most modern conventional engines and larger seat capacity, drastically reducing emissions compared to the smallest jets. And a turboprop of similar technology will beat a jet by 20-30% in consumption and emissions, thus making it more economically viable than a comparably sized jet when looking at efficiencies per passenger or per passenger/km. Replacing antieconomic jets with turboprops on regional and short-haul routes is a target achievable by mid-of-this decade. Moreover, these planes can also operate with SAF, reducing emissions even further.
4.3 PATHWAY #3: Create a clear regulatory framework for fleet renewals
It should not be economical to fly old and inefficient airplanes simply because they are already written off financially. Without a global carbon tax, governments should limit fuel consumption per seat mile, forcing older planes out of the skies. The same should happen with freight. In the past, older aircraft have been converted into freighters and thus keep polluting the air. We suggest limiting standards in carbon emissions per ton of freight.
The bulk of active commercial aircraft remains in the single-aisle narrowbody category – specifically, the Airbus A320 and Boeing 737 families. While the consolidation of air traffic during the pandemic has already ensured that the oldest “hogs” in global aviation are no longer flying (e.g., the MD-80s in North America), let’s not forget that as mentioned, even between the two latest generations of the best-selling single-aisle aircraft, there is a difference in fuel consumption per seat kilometer of up to 20%. Further incentivizing airlines over several years to switch from their 737 NG / A320ceo fleets to MAX/neo aircraft could already have a sizeable impact on overall emissions. The same applies to moving out older long-haul generations like the A330 Classic, A340s, and Boeing 767s. If you think this is far-fetched: it is precisely what the German government introduced in the automobile sector during the financial crisis of 2008/09 (and again recently with electric vehicles), where citizens were nudged to replace their old cars with more modern versions requiring less energy/fuel.
-
Clear guidelines for implementing decarbonization efforts in the next 20 years, all while being able to continue with longer-term investments such as into hydrogen.
Easy planning & implementation, as no significant logistics and infrastructure changes are required.
-
Easy planning & implementation with little to no investments in infrastructures needed to be made.
Easy planning & implementation, as no significant logistics and infrastructure changes are required.
-
Mitigation of risk between current solutions and longer-term innovations.
Full compatibility with existing products & go-to-market strategies.
Stimulation of production without requiring a multi-billion investment into brand-new designs.
-
Clear guidelines for planning and investment.
Historic opportunity to build infrastructure.
Significant leverage for positive public awareness of the oil & gas industry – from pollution to solution.
-
Better predictability of an otherwise complex market.
Clear investment cases to execute.
-
Executability of action on international, national, and regional levels.
High leverage for strategy and actions.
4.4 How the pathways will benefit stakeholders
5) Getting Into Action
With pathways and benefits outlined, what will need to get from theory to practice?
1| Political Incentives
Today, SAF production cost is, on average, between two and four times the cost of conventional fuel. Government support and incentives will be required until the industry matures and produc- tion costs drop. The same goes for an emission tax, of course. It is perhaps the most vital step in the chain, but one that airlines and airports cannot address on the free market without losing all com- petitiveness. Within Europe, it is an issue that needs to be discussed on the transnational level to generate an impact. On the production side, moving forward, the major challenge is mobilizing investments to develop multiple new large-scale facilities to increase SAF production so that additional demand can be met and production costs are reduced.
5.1 The cornerstones of implementation
2| Coalitions of the Willing
Historically, the aviation industry has yet to be successful in speaking with one voice. Despite institutions like IATA, different stakeholders have focused primarily on their own agendas. For a real uptick in decarbonization efforts, we must create a more open and hands-on mentality where airlines, airports, fuel providers, and manufacturers work together closely and visibly for the same goal globally.
3| Research & Development
Aside from longer-term technologies like hydrogen, more R&D has to be directed toward lowering the production costs of SAF and ways to scale production efficiently. It is the most effective solution for the next 20 years, and research activity and funding should reflect this. In these areas, there is still much potential to be unlocked in the next two decades.
4| Regulatory Support
An unclear regulatory situation is one of the reasons why investment into SAF is still relatively slow. In short: In many cases, we just don’t know which kind of SAF and which type of production method will qualify for a ‘sustainable’ label by authorities and regulators. This “limbo” situation slows innovation and must be solved quickly. A global certificate and framework system is required NOW to avoid spending billions on technical research and production plants without achieving the promised global impact in the required timeframe. Capital will only be available for technologies with the stamp of approval to yield future benefits.
5| Public Awareness
So far, aviation has missed to include the broad public in its strategies to make flying “greener.” Some questionable greenwashing activities, especially by airlines, have not helped us in the past as well. As an industry, we need to become better at making people aware that we are working hard on decarbonizing flight and that even today, passengers can already play an active role in de- creasing their carbon footprint when they fly. The implementation of information on CO2 emissions in the Google Flights search is an excellent example of how to raise more awareness on this. Still, a tech company did it, not the aviation industry. Airlines adding a “Green” option or class at booking is another excellent example of how we can upsell a SAF or offset option already in the booking process, just like you add luggage. Why doesn’t every airline do this? If they fly a GTF-equipped aircraft or CO2-efficient turboprop on a particular route, why don’t they also advertise this in the booking process?
5.2 Recommended next steps for every stakeholder
-
Engage more proactively in political affairs to resolve pending SAF questions. “Lighthouse” projects, such as Virgin Atlantic’s transatlantic SAF trial, can be an intelligent first step.
Double down on committing to purchase SAF and create a strategy for scaling blends.
Communicate your strategy and actions more transparently to the passengers (see the example from the booking process).
Follow a path of less: burning less, using less, emitting less – by rethinking business and operational models and offering more innovative products that are more “door-to-door.”
-
Secure availability of SAF, with supply still being limited today.
Engage more proactively in political affairs to resolve pending SAF questions. Progress in this field will also benefit the airport’s relationship with the public.
Form coalitions with other airports and/or airlines to speak with one voice.
-
Engage more proactively in political affairs to resolve pending SAF questions. That includes the creation of more “lighthouse” projects as well as discussions on blends and certification.
Reassess turboprop demand.
-
Grow through further pilot projects.
Engage with governments and regulators to address specific needs and stimulants, and work with other aviation stakeholders to consolidate unified messages.
-
Introduce incentives for investment in production facilities. Increasing quotas of SAF percentages would create such an environment.
Introduce limits on fuel consumption and/or emissions per seat mile, forcing older planes out of the skies.
Create incentives to reintroduce more turboprops on regional routes.
Align at the international level (EU, North America, Asia, UN, etc.) to address the global value chain. A single-country approach to an international industry will not move the needle. Most of the global commercial aircraft fleet is lessor-financed, and these financial companies will not take significant risks.
We hope that in the second half of the 21st century, we will see aviation overcome the next frontier, with technologies like hydrogen or fuel cells becoming mainstream for all aircraft sizes and ranges.
Yet we believe it is our responsibility today to accelerate a drastic reduction in CO2 emissions and make a joint effort to implement the maximum reduction of aviation’s greenhouse gas emissions with the limitations of physics as they apply today.
We are confident that the three pathways can be implemented quickly and that we have enough opportunities to reduce emissions effectively until 2050. Let us start bridging the gap today. We have the ingredients already.
6) About Sustainable Aero Lab
The vision of the Sustainable Aero Lab is to make climate-neutral aviation a reality.
Founded in December 2020, Sustainable Aero Lab is the world’s leading fast-track program dedicated to accelerating companies in sustainable aviation. We do this by globally identifying and bringing together promising startups with experienced mentors and investors in live sessions and one-to-one coaching, opening doors and finding customers, new projects, and partners. Participation in the Lab is free to all startups, and admissions are rolling without deadlines.
In the first two years since our inception, over 40 companies have been accelerated in the pro- gram, with numerous collaborations and investments made. Our mentors are highly recognized individuals from aviation, industries, and venture capital who are motivated to share their experience and help companies build a new ecosystem of sustainable aviation.
In addition, Sustainable Aero Lab serves as a platform to publicly discuss and promote zero-emission aviation technologies, including developments such as SAF and hydrogen, without any specific preconditioning or sponsors.
If you want to learn more and get in touch with us, visit www.sustainable.aero and follow us on LinkedIn.
7) About the Authors
Nico Buchholz has a background in aeronautical engineering and management. He has over 30 years of international experience covering all continents, mainly in the airline and aerospace industries. Nico worked on the advisory teams of basically all airframe and engine manufacturers and took a key role in pushing several (game-changing) aircraft designs like the CSeries (A220), Embraer E2, A320NEO, A350, 787, 747- 8, and 777X. He was responsible for the Lufthansa fleet for 15 years and purchased and sold over 1,000 aircraft in his career. Nico held other senior functions in Airbus, Rolls-Royce, and Bombardier, on the boards of two leasing companies, and has assisted several airlines globally on fleet matters. Today he is a board member at several aviation-related companies and a mentor at the Sustainable Aero Lab.
Nico Buchholz
Bjorn Fehrm is an airliner analyst at Leeham Company, focusing on technical and economic evaluations for airlines, lessors, OEMs, and investors. He‘s an aeronautical engineer and ex-fighter pilot from the Swedish Air Force, where he flew the Draken and worked on the Viggen and Gripen programs. Bjorn has worked at SAAB with the Gripen program in the area of counter-measures. He has piloted 15 aircraft types and is the developer and expert on Leeham Company’s Aircraft Model, which enables a complete performance analysis of aircraft from aerodynamic data to operational costs. Bjorn is also a mentor at the Sustainable Aero Lab.
Bjorn Fehrm
Lukas Kaestner is a co-founder and Chief Commercial Officer of the Sustainable Aero Lab. In addition, Lukas is the Chief Development Officer of the ZAL Center for Applied Aeronautical Research in Hamburg, one of the world’s most renowned innovation centers for commercial aviation. Lukas has been an aviation enthusiast since childhood and has been involved in the industry for many years. Among other roles, he has chaired the Crystal Cabin Award Association, the world’s most recognized award for aircraft interiors and passenger experience, and served as Head of Marketing, PR & Events at the Hamburg Aviation Cluster.
Lukas Kaestner
Stephan Uhrenbacher is a serial entrepreneur and works as a coach to entrepreneurs. He has founded and scaled numerous internet companies such as Qype and 9flats.
Stephan shifted his focus to sustainability in 2010 when he co-founded Avocadostore, the German marketplace for eco-friendly consumer goods. He is a startup mentor at the Creative Destruction Lab in Toronto (AI) and Oxford (Climate). Along with advancing sustainable aviation through the Sustainable Aero Lab, Stephan is a globally sought-after coach and sparring partner to entrepreneurs.
Stephan Uhrenbacher
Mario Vesco is Venture Manager at the Sustainable Aero Lab, where he identifies, assesses, and works with startups holding the potential of meaningfully contributing to aviation sustainability. Mario is an experienced expert in commercializing novel technologies and innovations in aerospace, including drones. He has been working in aerospace since 2001. Before joining the Sustainable Aero Lab, Mario worked as a consultant for corporates, SMEs, and startups, bridging the gap between technology and business creation. He holds an MSc in Aerospace Engineering from the University of Rome (IT), an MBA from Nyenrode Business University (NL), and participated in the Ph.D. program of the Delft University of Technology (NL).
Mario Vesco